Mitochondria as the ultimate drivers of health and disease
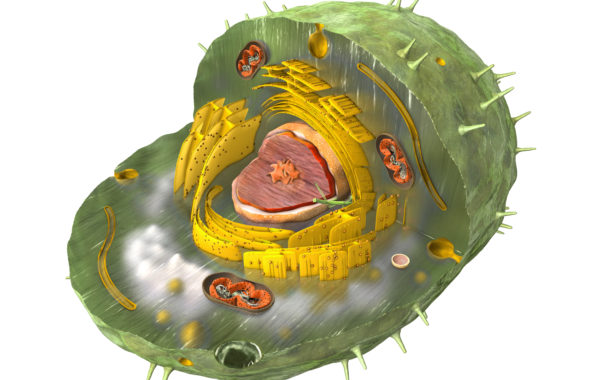
Our mitochondria are the ultimate orchestrators of our cellular health. The evolutionary origin of our cells is fascinating: they have a hybrid nature stemming from a dual genome.
THE ORIGIN of the hybrid nature of our cells lies far back in history – around 2.1 billion years to be exact – when the first Ice Age occurred. Before this, the Earth’s atmosphere was devoid of molecular oxygen, but dominated by carbon dioxide (CO2) and methane gas (CH4). The CO2 was a result of volcanic activities in the Earth’s crust, while the CH4 was produced by the ubiquitous archaea – a largely anaerobic life form – which converted CO2 into CH4 [1].
When the global ice sheet melted, the O2 concentration in the atmosphere began to rise exponentially, most likely as a result of cyanobacteria metabolising CO2 into oxygen via photosynthesis. This is clearly evidenced in oxide bands in iron sediments from that time [2].
The existence of the archaea was now threatened. Evolutionary biology has established that the most likely explanation for the development of eukaryotes – cells with a nucleus and organelles – was that the archaea engulfed proteobacteria, which already at that time had a functioning electron transport chain, so could metabolise oxygen [3]. These bacteria enabled the archaea to survive in areas where oxygen levels were high, and were the predecessors of our mitochondria.
Mitochondria still possess many morphological and biochemical features of their bacterial ancestors, including a double membrane, a circular genome, the absence of histones, and the ability to replicate independently of the nucleus [4]. Our cell’s nucleus with its DNA stems from the archaea, while the mitochondria have their own separate genome. This dual genome is a large factor in our health and disease.
Evolutionary advantage
To understand why, we first need to look at what the evolutionary advantage was for these bacteria of living within an anaerobic host? Certainly one can imagine that this would at that time have ensured their survival in areas where oxygen levels were still low, but there is another fascinating reason.
The generation of ATP (adenosine triphosphate) using oxygen produces large numbers of free radicals. These would damage the DNA of the cell nucleus when it divides, as it is not covered by a protective envelope during replication. Cyclin D1 – one of a family of proteins that are active at different times in the cell cycle – appears to inhibit the mitochondria during the S phase when DNA is replicated [5].
At this point the archaea’s anaerobic energy generation – glycolysis – is used instead as no oxygen is required, and therefore no oxygen radicals are produced.
This switching from one form of energy generation to the other is an ancient programme that our cells still carry within them: the archaeal genome is largely responsible for replication and repair, while the mitochondria are responsible for high-intensity energy production and many of the most sophisticated aspects of cell signalling [6].
The interaction of the symbionts and their host
Before thinking about how this switching process can go wrong – which lies at the heart of mitochondrial dysfunction – we should look at the two very different forms of energy generation, because this is also key: intramitochondrial, and cytosolic.
When ATP is produced in the intracellular fluid (cytosol) via glycolysis, one molecule of glucose generates a net yield of 2 ATP, whereas inside the mitochondria, the net yield via the electron transport chain (ETC) is far greater: approx. 30 – 36 ATP [7] from one molecule of glucose (exact figures vary), and even higher from fats, e.g., one molecule of oleic acid generates about 146 ATP.
But it’s not just 2 versus 36 (or 146). We have around 1,500 mitochondria in each cell on average (though this varies hugely – erythrocytes are the only cells that have none), and thousands of electron transport chains within each mitochondrion [8]. So the energy generation our cells are capable of if oxidative phosphorylation (energy production along the ETC) is fully functional is infinitely more efficient than cytosolic energy generation.
This is why the symbiosis of these two organisms – archaea and proteobacteria – was so fundamental to evolution: the huge energy production possible along the ETC enabled the development of multicellular life.
However, if constant cellular repair processes are necessary, the archaeal genome is upregulated, as the genes exposed during cellular repair and replication would be damaged by the oxygen radicals being fired off continually along the ETC.
An additional reason is that glycolysis produces building blocks for the synthesis of new macromolecules essential to cellular repair processes (such as nucleic acids, lipids and proteins) [9]. Chronic inflammation mirrors exactly this scenario: cells are constantly being sloughed off and need replacement. It is therefore part of our natural programming for the archaeal genome to be upregulated and mitochondrial activity to be downregulated during chronic illness that involves any kind of cellular replacement and repair.
Mitochondrial dysfunction
When the mitochondria shut down there is clearly going to be a major energy production problem. What can cause mitochondria to shut down or cease their normal function? They may lack some of the enzymes or co-factors required for the different complexes in the electron transport chain. They may lack sufficient antioxidants – especially glutathione – to quench either endogenous or exogenous free radicals. Heavy metals or other xenobiotics may be adducted to the cellular or mitochondrial membrane. Defects in detoxification may prevent toxins from being eliminated, causing blockages in normal cellular function. And as mentioned at the outset, infection or inflammation of any kind – due to the cellular turnover and therefore repair that it involves – leads to a switch to greater cytosolic energy generation and downregulation of the mitochondria [10].
The mitochondria are also responsible for releasing cytochrome c whenever cells need to apoptose (self-destruct). This will not happen if this switch has occurred and the archaeal genome is running on automatic: cell proliferation is a possible consequence due to the inability of cells to undergo apoptosis – obviously a highly deleterious aspect of this shift, quite apart from all the other issues related to mitochondrial dysfunction. The mitochondria have such myriad functions. Apart from the energy they produce and the cell-cell communication just touched on, they are intimately interlinked to the T-helper 1 and T-helper 2 cells (Thl /Th2 arms) of our immune system, and the nitric oxide that is our primary intracellular defence against pathogens [11].
Mitochondria appear to have their own mitochondrial nitric oxide synthase that acts as a metabolic regulator, but if our antioxidant systems are overwhelmed, nitric oxide gas (NO) modulation goes awry, and the Th2 arm of the immune system is upregulated to compensate. This is a pattern that often accompanies mitochondrial dysfunction, and means that intracellular pathogens cannot be fought off successfully.
The first step of heme synthesis also takes place in the mitochondria using aminolevulinic acid synthase, so if the mitochondria are down, heme cannot be properly synthesised [12]. This does not just affect our haemoglobin production. Heme is rate-limiting in numerous proteins in the body: myoglobin, neuroglobin, cytochrome P450, the cytochromes of the electron transport chain, and multiple others [13]. Our mitochondria are also key to cellular detoxification. Beta oxidation takes place in the mitochondria, so fatty acids will not be broken down efficiently; the first step of the urea cycle is in the mitochondria, so the excretion of protein will be disrupted [14]. And monoamine oxidase is located on the outer mitochondrial membrane, which catalyses the breakdown of the monoamines, whether dopamine, serotonin, noradrenaline or adrenaline, so these neurotransmitters will be dysregulated [15].
Even excess calorie intake can throttle oxidative phosphorylation, as a high proton-motive force (which high calorie intake produces) leads – if unabated – to the generation of more reactive oxygen species than we have antioxidants to quench. The mitochondria downregulate as a result, to protect the cell from destruction [16].
Mitochondrial markers
A number of tests can be used to measure mitochondrial versus glycolytic energy production, as well as many other parameters. Please see our Mitochondrial Testing Page to find out more about the mitochondrial tests that AONM offers.
References
- Woese CR., Stackebrandt, E., Macke TJ, Fox, GE. A phylogenetic definition of the major eubacterial taxa. Department of Genetics and Development, University of Illinois, Urbana 61801, USA; https://pubmed.ncbi.nlm.nih.gov/11542017/
- https://news.wisc.edu/ancient-rocks-record-first-evidence-for-photosynthesis-that-made-oxygen/
- Zimmer, C. (2009) Science 325_666, On the origin of eukaryotes; https://science.sciencemag.org/content/325/5941/666
- Krysko, D., et al. Emerging role of damage-associated molecular patterns derived from mitochondria in inflammation, Trends in Immunology, April 2011, Vol. 32, No. 4; https://pubmed.ncbi.nlm.nih.gov/21334975/\
- Martinez-Diez M, et al. Biogenesis and Dynamics of Mitochondria during the Cell Cycle: Significance of 3’UTRs. PLoS ONE 2006, 1(1): el 07; https://journals.plos.org/plosone/article?id=10.1371/journal.pone.0000107
- Meyer, R. Cell Symbiosis Therapy, A Revolutionary New Approach to Chronic Disease, 2009, Prinz-Druck, Idar-Oberstein, Germany; https://www.ncbi.nlm.nih.gov/pmc/articles/PMC7116391/ Ryan, Dylan G et al. “TCA cycle signalling and the evolution of eukaryotes.” Current opinion in biotechnology vol. 68 (2021): 72-88. doi:10.1016/j.copbio.2020.09.014
- Rolfe, D. F., Brown, G. C. (1997). Cellular energy utilization and molecular origin of standard metabolic rate in mammals. Physiol Rev. 77(3):731-58, https://pubmed.ncbi.nlm.nih.gov/9234964/; Berg JM, Tymoczko JL, Stryer L. Biochemistry. 5th edition. New York: W H Freeman; 2002. Section 18.6, The Regulation of Cellular Respiration Is Governed Primarily by the Need for ATP,https://www.ncbi.nlm.nih.gov/books/NBK22448/
- Voet, D., et al (2006). Fundamentals of Biochemistry, 2nd Edition, John Wiley and Sons, Inc.; https://blackwells.co.uk/bookshop/product/Fundamentals-of-Biochemistry-by-Donald-Voet-Judith-G-Voet-Charlotte-W-Pratt/9780471214953
- Calderon-Montano, JM et al., 2011. Role of the Intracellular pH in the Metabolic Switch between Oxidative Phosphorylation and Aerobic Glycolysis – Relevance to Cancer. http://www.webmedcentral.com/article_view/1716
- Kremer H, MD, The Secret of Cancer: Short-Circuit in the Photon Switch. Townsend Newsletter, August/September 2007
- Bogdan, C. (2001). Nitric oxide and the immune response. Nat. Immunol. 2(10): 907 – 16
- Ajioka, RS, et al. (2006). Biosynthesis of heme in mammals. Biochimica et Biophysica Acta 1763 (2006) 723-736
- Rochlitz, S. (2010) Porphyria: The ultimate cause of common, chronic & environmental illnesses (self-published)
- Miles, B. The Urea Cycle. https://www.tamu.edu/faculty/ bmiles/lectures/urea.pdf
- Zwart, K et al. (1980). Development of Amine Oxidase¬Containing Peroxisomes in Yeasts During Growth on Glucose in the Presence of Methylamine as the Sole Source of Nitrogen Arch. Microbiol. 126, 117-126; https://pubmed.ncbi.nlm.nih.gov/7192080/
- Mabalirajan, Ulaganathan, and Balaram Ghosh. “Mitochondrial Dysfunction in Metabolic Syndrome and Asthma.” Journal of Allergy 2013 (2013)
Contact details
03331 210 305
Administration:
St. John’s Innovation Centre, Cowley Road, Cambridge CB4 0WS